Introduction
Throughout history head hair has always been of enormous social significance to humanity; the quality of our hair is synonymous with how we feel. Healthy hair is a sign of youth and wellbeing; it instils confidence and is a source of pride. It comes as no surprise then that we give it our undivided attention, it is washed and groomed regularly and we feel justified in spending whatever is needed to maintain and enhance its appearance. Consequently, the global market for hair care related products is currently estimated to be worth about $42bn per annum and is forecasted to be almost $58bn by 2015.1,2
Environmental sunlight is perceived to be of benefit to our general health, indeed Vitamin D biosynthesis from sunlight exposure is essential. However, it can also be very damaging due to the energy present in solar ultraviolet radiation (UVR). UVR can be categorized into three different wavelength bands, namely UV-C (200-280 nm), UV-B (280-320 nm) and UV-A (320-400 nm).3 Of these, UV-B is the most detrimental as it contains the highest energy;4 it is absorbed by the ozone layer, but anthropogenic depletion of the stratospheric ozone layer means that more of this high energy radiation now reaches the earth’s surface where it is accompanied by the less energetic, but also harmful, UV-A band.5,6
Although sunburn, ageing and wrinkling of skin brought about by exposure to UVR is well documented, less attention has been paid to the fact that it can have an equally adverse effect on hair. UVR damages hair in several ways: it penetrates the cuticle leading to the generation of reactive oxygen and nitrogen species,7 leading to photo-oxidation of keratin fibres by breaking the disulfide crosslinks between the amino acid backbones, whilst also promoting decomposition of the natural melanin pigment and lipids.8,9 This results in overall damage of the protein ultra-structure leading to oxidative bleaching of both natural and artificial colours in the hair.10 Furthermore, this can cause loss of tensile strength, increased brittleness and damage of hair texture.
Evaluating UV damage on human hair
Many factors can influence the analysis of damage to hair caused by UVR; moisture, condensation humidity, and temperature all have a significant influence on photo-oxidation, as does the sample being evaluated (pigmented? chemically treated? etc.). Spectral distribution of the irradiating light is also important as certain wavelengths are capable of cleaving specific chemical bonds, and the shorter the wavelength the greater the energy of the radiation; presentation angle incident to the light source is also a critical factor.
Typically, evaluation of hair tresses takes place in a controlled chamber at a constant temperature of 25 °C and 65% relative humidity; samples would be positioned 10 cm away from the light source. Irradiation would typically include the wavelength range 280-320 nm (~0.15 mW cm-2) for UV-B and 320-400 nm (~0.50 mW cm-2) for UV-A.9 Tresses would be rotated constantly to assure uniform exposure. Any changes to the hair would be evaluated at intervals over a period of several days; various parameters can be assessed to evaluate changes that have occurred as a result of UVR exposure:
- Hair colour: hair tresses can be measured before and after UV irradiation using a spectrophotometer. Colour changes can be calculated using the CIELab system (colour difference, lightening, yellowing). This system is the most appropriate for evaluating changes in natural or applied colour, but is not reliable in terms of assessing structural damage to the hair fibre.
- Tensile strength: breakage resistance (in terms strain and extension at break) of single fibres is evaluated using an Instron testing apparatus under controlled atmospheric conditions. This is repeated for many fibres in the hair tress and related to each fibre’s cross-sectional area to calculate Young’s modulus. The main disadvantage with this technique is the number of individual measurements and the time involved to achieve a reliable result.
- Tryptophan concentration: concentration of the amino acid tryptophan (Trp), present in keratin, is known to reduce when hair is exposed to UVR, and the concentration of Trp can be measured using fluorescence emission spectroscopy.11 Trp measurement may be a useful early indicator of photo-damage to hair, but has not been sufficiently been demonstrated to be a reliable indicator of absolute damage.
- FT-IR spectroscopy: It is known that UVR promotes photolysis of disulfide bonds present in cystine residues in hair fibres, which are fundamental to the cross-linked structural nature of hair proteins.12-14 These disulfide bonds may be broken in a number of chemical ways, such as in the process of bleaching, in the formation of a “permanent wave” hairstyle, or as a result of exposure to UVR (Figure 1). Attenuated Total Reflectance Fourier-Transform Infrared (ATR FT-IR) spectroscopy offers many advantages in assessing hair damage: there is no requirement for sample preparation as the tresses can be presented directly to the instrument; measurement and analysis is rapid; and the technique reliably assesses damage to the hair.
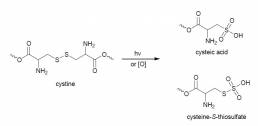
Figure 1. Structure of cystine residues on hair and possible oxidative degradation products.
Because of these advantages, we use ATR FT-IR spectroscopy to assess damage to hair fibres from UVR exposure. Numerous spectra are collected, with sample points taken at various sections of the hair tresses, and also by rearranging the fibres, and offering different fibres from the same tress to the sample holder. All spectra are normalised to the reference peak of the amide N-H stretch at ~1600
cm-1, which is believed to remain unaffected in intensity across the samples. Figure 2 (left) shows that bleached hair, has a strong peak in the region of 1042 cm-1, which is characteristic of a S=O stretch present in cysteic acid, and a broader stretch also attributed to S=O, characteristic of a sulfonate S=O stretch (1175 cm-1), which occur as a result of chemical cleavage (oxidation) of the cystine bond. Similarly, Figure 2 (right) shows that these characteristic peaks are present as a result of photolysis of the cystine residues, and hence UVR related damage to the hair fibres.
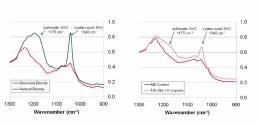
Figure 2. FT-IR Spectra in the region of 1300-900 cm-1: natural blonde and bleached blonde hair (left); natural blonde and natural blonde after UVR exposure (right).
Current UV absorber technology
A major challenge facing the cosmetics industry has been the development of formulations that confer adequate protection against UV damage without compromising hair appearance. Such protection is typically provided by the inclusion of UV filters more commonly known as sunscreens. The ‘colour protection’ market alone is worth about $10bn per annum.15 The majority of sunscreens currently found in shampoos and conditioners can be classified as either organic or inorganic chemicals which act by absorbing and reflecting/diffusing UVR, respectively.16
Organics absorb UV light over a particular wavelength range depending on their chemical structure. This functionality is facilitated by a chromophore consisting of a conjugated system comprising p-orbitals with delocalised π-electrons with alternating single and multiple bonds between the different atoms.16 Absorbed energy from UV light results in electrons transferring to a higher energy orbit in the molecule; the sunscreen active molecule thus enters what is known as a higher energy or so-called excited state, this energy may then be released as heat in the form innocuous infrared energy through deactivation of the excited state.17 At present, there are eight different families of organic filters that have FDA approval for inclusion in cosmetic products, these comprise six UV-B absorbers (salicylates, PABA esters, cinnamates, benzimidazoles, cyanoacrylates, benzophenones) and two UV-A absorbers (anthranilates, dibenzoylmethanes) as shown in Table 1.
UV-B filters are relatively photo-stable and efficient, and have thus been included in marketed products for several years, the challenge however lies with developing suitable UV-A filters; this is because UV-A filters are generally not photo-stable and rapidly degrade upon UVR exposure, resulting in a marked reduction in their efficacy.17,18 This can also lead to the generation of dangerous radical species which in turn interact with biological matrices bringing about an overall reduction in hair quality.7,9,10 UV-A may be further subdivided into UVA-1 (340-400 nm) and UVA-2 (320-340 nm). Of the UV-A filters available, butyl methoxy dibenzoylmethane (avobenzone) is the most widely used;15 it absorbs maximally in the UVA-1 range, but is not photo-stable requiring terephthalylidene dicamphor sulfonic acid (ecamsule) for stabilisation in formulation.17,20 Ecamsule is a broad-spectrum UV filter that absorbs across both the UV-B and UVA-2 regions thus giving a combined formulation that provides protection over the entire UV-A range.21 There has, however, been notable disparity in the regulatory approval of ecamsule; although it has been available in Europe since 1991 it only received FDA approval in 2006.21
In general, where sunscreens are concerned this pattern also holds true: in 2005 there were only 16 individual sunscreens approved for use in the US whereas in Europe, Australia and Canada the figure was significantly higher, 28, 26 and 21 respectively.22 As of 2011, the FDA sunscreen monograph still listed only 16 UV filters.23 This disparity is due to the fact that the FDA tends to treat sunscreens as new drug applications which require a more rigorous validation whereas outside the US new sunscreens are generally treated as cosmetics with the approval process having less stringent criteria. Although the FDA has recently introduced certain procedures designed to fast track cosmetics to market progress remains slow.22
Of the inorganic group, titanium and zinc oxide are probably the best known and they work in combination with organic filters to provide protection in the UVA-1 range. However these are large, relatively photosensitive particles and absorb light from the visible region which leads to an undesirable white appearance on the treated surface.17,18 While it may be argued that the generation of nanoparticles of titanium and zinc powders may have facilitated user acceptance of these filters, such nanoparticles may be more easily absorbed into the body. Therefore concerns have been raised about the potential long term toxicity this may pose for the consumer.24 Furthermore, it has been shown that the smaller particle size results in a shift in absorbance away from the longer UVA-2 range towards the UV-B range, consequently decreasing the overall efficacy of such formulations.17
Although many sunscreens have been formulated for use in hair shampoos, conditioners and serums, their efficacy is often questionable; a sunscreen that is highly effective on skin may not necessarily be effective on hair. When applying hair care formulations containing UV filters there is limited contact time between the hair and the active ingredients to enable deposition/adsorption onto the hair. Many UV filters have limited or no affinity for the hair substrate; oil-soluble UV filters are particularly problematic and their deposition onto the substrate is often difficult and very formulation-dependent; water-soluble UV absorbers that do have an affinity for the hair can often be easily washed off the hair during the rinsing steps of the application. Inorganics have no affinity for hair. This is an extremely important issue as it may determine the efficacy of the UV filter in providing efficacious UV protection to the hair.
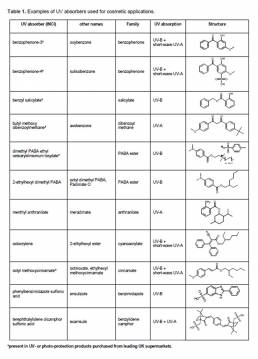
Experiments using benzophenone-3, benzophenone-4, 2-ethylhexyl dimethyl PABA, and phenylbenzimidazole sulfonic acid demonstrated that none of the UV filters used diffused through the hair fibre and the distribution of the actives on the hair surface varied greatly, dependent upon the delivery formulation.25 Overall efficacy of the UV filters was significantly influenced by the complex interactions between the active, the delivery vehicle and the substrate. Various attempts have been made to improve the delivery of lipophilic UV absorbers using cationic modifications to electrostatically bind to the negative sites in the hair fibre: One method improves application properties by using cationically charged nano-vesicles prepared by high-pressure homogenization of a phospholipid dispersion;26 other work showed that the cationically modified UV absorber cinnamidopropyltrimonium chloride (CATC), displayed significantly higher deposition onto the hair from a rinse formulation, in comparison with the non-ionic octyl methoxycinnamate (OMC), which showed only minimal deposition.9
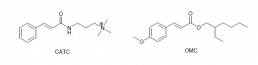
Particular challenges exist in formulating with sunscreens in hair care:
- For shampoos and conditioners (rinse-off), oil-soluble UV filters require emulsification; water-soluble UV filters are more easily incorporated, but may have pH stability issues.
- For styling products, water-soluble UV filters can have a salt-thinning effect; oil-soluble UV filters require emulsification and can have a significant impact on viscosity.
- For hairsprays, large amounts of ethanol enable easier formulation, but deposition is often as droplets and the product does not provide a continuous film, hence protection is not uniform.
- Some UV filters are powdery substances with an off-white appearance and may crystallise on the hair surface when the delivery solvent has evaporated.
The future of hair protection against UV
Although several sunscreens are available that may be included in formulations to protect hair from UV damage, issues concerning their relative stability, efficacy, toxicology and lengthy FDA approval procedures is making their continued development a sizable challenge to the cosmetics industry. Perhaps the industry should be more focussed on exploiting natural alternatives in a sustainable way. Several natural extracts and products are listed in the CosIng database as ‘UV absorbers’ (Table 2), however, the active ingredients are not disclosed and there is very little literature on the specific mechanism of action in cosmetic products. Some of these products are found in skin care products, but not have been found in commercially available hair care products by the authors.
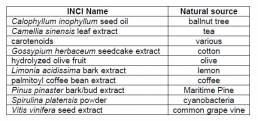
Table 2. Naturally derived UV absorbers listed in CosIng database.
Mother Nature synthesises complex and useful chemistry, we should understand what this chemistry can do for us and use it to our advantage. Such an approach for UV hair care products may have fewer constraints attached if reliable source, low toxicity, efficacious actives can be identified.
Of the natural alternatives that exist, polyphenols from fruits and vegetable sources may hold the greatest promise. Of the several thousand structures known, the flavonoid class of pigments constitutes the largest group, including the UV absorbing flavone, flavonol, flavanone and isoflavone classes; flavones and flavonols are universal in occurrence whereas the other classes have a more restricted distribution.
The flavonoid structure imparts intense absorption in the UV spectrum and the conjugated system provides a very similar functionality to that seen with the organic class of UV filters.3,16 In fact the UV-B attenuation properties of flavonoids are well established with strong evidence available to suggest that this is a primary ecological function of these compounds in the plant.29-31 However their potential efficacy these compounds as broad spectrum UV filters has been largely overlooked. Furthermore, these compounds are well known potent radical scavengers and antioxidants.32-34 In this way such compounds could provide a dual function in hair sunscreens; they may filter UV-B and UV-A radiation effectively whilst maintaining stability, and secondly scavenge any reactive oxygen and nitrogen species generated by UV damage.
Flavonoids are relatively water soluble which makes them easy to incorporate into formulations, although lipophilic derivatives are also known to occur.35,36 Plant extracts rich in different flavonoids may thus be ideal candidates for inclusion in hair sunscreens for the following reasons:
- They have the potential to be broad-spectrum UV filters
- They are relatively resistant UV irradiation
- They have varied solubility and could be included in both water and oil based formulations
- They are natural products with putative safety; they are key components of our diet through daily consumption of fruit and vegetables and thus should be attractive to the end user
- They may be cost effective. They are widely distributed throughout the plant kingdom and can thus be easily obtained from the several waste streams processed by the food industry
These are all key criteria in the successful development of efficient sunscreens. The cosmetics industry is gradually beginning to realise this. Although the development of natural hair care products is still very much a niche area there are some products containing natural sunscreens.
However, most of these products are designed for providing UV skin protection, and research is needed to identify actives and develop delivery methods to achieve deposition onto hair. Potential challenges such as achieving good wash fastness of such natural product containing formulations will also need to be overcome.
Nevertheless, regardless of the nature of the developed formulation, in order for such a strategy to be attractive, natural products should be resourced in a sustainable way; certainly a combination of the widespread occurrence of certain classes of flavonoids and large waste streams processed by the food industry may offer potential in this regard and in this way materials may be sourced that do not compete with land crops for food. In the current climate consumer attitudes are shifting towards more natural and greener based technologies, therefore now more than ever is the time ripe for developing such a strategy.
References
1. www.bi-me.com/main.php?id=46314&t=1, Accessed 8 Feb 2012.
2. Trans World News, 2011. Global Haircare – Market Research Report.
3. Cockell CS, Knowland J. Biol. Rev. 1999, 74, 311-345.
4. Harborne JB, Williams CA. Phytochemistry 2000, 55, 481-504.
5. Blumthaler M, Ambach W. Science 1990, 248, 206-208.
6. Hester SA. Pharmacist’s Letter/Prescriber’s Letter 2007, 23, 230806.
7. Herrling T, Jung K, Fuchs J. SÖFW-Journal 2007, 8.
8. Jung K, Herrling T, Blume G, Sacher M, Teichmüller D. SÖFW-Journal 2006, 7.
9. Gao T, Bedell A. J. Cosm. Sci. 2001, 52, 103-118.
10. www.touchbriefings.com/pdf/846/Maillan.pdf, Accessed 2 Feb 2012.
11. Pande CM, Jachowicz J. J. Cosm. Chem. 1993, 44, 109-122.
12. Signori V, Lewis DM. Int. J. Cosm. Sci. 1997, 19, 1-13.
13. Signori V, Lewis DM. Macromol. Symp. 1997, 119, 235-240.
14. Signori V, Lewis DM. J. Cosm. Sci. 2004, 55, 95-113.
15. www.icis.com/Articles/2008/05/14/9123943/isp-lanches-hair-dye-retention-technology.html, Accessed 5 Feb 2012.
16. Kimbrough DA. J. Chem. Ed. 1997, 74, 51-53.
17. Forestier S. J. Am. Acad. Dermatol. 2008, 58, S133-S138.
18. Gonzalez S, Gilaberte Y, Philips N, Juarranz A. Open Dermatol. J. 2011, 5, 6-14.
19. Armeni T, Damiani E, Battino M, Greci L, Principato G. Toxicology 2004, 203, 165-178.
20. Paris C, Lhiaubet-Vallet V, Jiménez O, Trullas C, Miranda MÁ. Photochem. Photobiol. 2009, 85, 178-184.
21. Fourtanier A, Moyal D, Seité S. Photodermatol. Photoimmunol. Photomed. 2008, 24, 164–174.
22. pubs.acs.org/cen/coverstory/83/8315sunscreens.html, Accessed 1 Feb 2012.
23. FDA. Guideline for Industry. Enforcement Policy-OTC Sunscreen Drug Products Marketed without an Approved Application, 2011.
24. Lai JCK, Lai MB, Jandhyam S, Dukande VS, Bhushan A, Daniels CK, Leung SW. Int. J. Nanomedicine 2008, 3, 533-545.
25. Bernhard P, Giesen M, Hollenberg D, Hubbuch M, Kalhoefr V, Maier HE, Martin V, Munzing HP, Oelschlager T, Schwan A, Sperling K, Tenningkeit J. J. Cosm. Sci. 1993, 15, 181-199.
26. Zulli F, Suter F, Birman M. DCI, 1996, 46-48.
27. Georgalas A. Cosm. Toiletr. 1993, 108, 75-79.
28. Harborne JB. In: Dey PM, Harborne JB (Eds.) Methods in Plant Biochemistry, Vol. 1. Academic Press: London, 1989.
29. Li J, Ou-Lee T-M, Raba R, Amundson RG, Lasta RL. The Plant Cell 1993, 5, 171-179.
30. Lois R. Planta 1994, 194, 498-503.
31. Solovchenko A, Schmitz-Eiberger M. J. Exp. Botany 2003, 54, 1977-1984.
32. Burda S, Oleszek W. J. Agric. Food Chem. 2001, 49, 2774-2779.
33. Lee Y, Howard LR, Villalón B. J. Food. Sci. 1995, 60, 473-476.
34. Miller AL. Alt. Med. Rev. 1996, 1, 103-111.
35. Wollenweber E, Graven EH. Fitoterapia 1992, 63, 86.
36. Williams CA, Harborne JB, Greenham JR, Grayer RJ, Kite GC, Eagles J. Phytochemistry 2003, 64, 275-283.